For example, from SAE paper J357 "Physical and Chemical Properties of Engine Oils":
High-shear-rate viscosity measured at 150 °C and reported in mPa·s (centipoise) is widely accepted as a rheological parameter which is relevant to high-temperature engine performance. In particular, it is generally believed to be indicative of the effective oil viscosity in high-shear components of an internal combustion engine (for example, within the journal bearings and between the rings and cylinder walls) under severe operating conditions.
The bold yellow text in that statement are some pretty qualifying statements that indicate that there is not complete agreement among the experts. And those are some dudes with some really big heads! They even have really big names. For example - a "tribologist" is a scientist or engineer that studies the science of wear and friction.
So what is the average enthusiast like you or I supposed to do? .
What I did was read a ton of papers and articles to form a basic understanding of the principles involved so that I would be reasonably educated on the topic, allowing me to form at least semi-educated opinions when deciding what was best for my engine. I also built my own engine oiling system for my LS2 by assembling a collection of quality aftermarket parts including cooler, accumulator, remote filter, and custom plumbing.
So that's what I'm going to document here in hopes that you may find it useful. The bottom line is - I do not, in any way, claim to be an expert in this field, and some of what follows will unavoidably be opinion or educated guess. To paraphrase a friend of mine, "Oil...It's that damn complicated!"
So with that said, I'm first going to tackle an introduction to engine oil; and then finish with a look at the components that make up a quality oiling system - from pan to pump to accumulator and back.
Oil
What is oil?
Engine oil is not just a liquid - it's actually a "dilute polymer solution". What the heck does that mean? A polymer is a chemical compound made up of a long chain of repeating molecules. It means oil is a whole bunch of different chemical components and molecules combined is specific ways to create a sort of "liquid machine". Think of it like a whole bunch of tiny, microscopic molecular machines combined in long links like a bicycle chain.
Why is it so complicated?
The science of engine oiling is, in part, complicated because oil has so many important functions in the modern internal combustion engine. Ask someone what oil is for, and they usually get one or two from the list, but rarely all.
The purpose of engine oil is to:
- lubricate moving parts and protect against wear
- cool
- seal
- clean and maintain cleanliness
- provide necessary hydraulic action (e.g. hydraulic valve lifters)
- protect against corrosion
Basic oil composition
In order to be able to do all that, over a wide range of operating conditions (esp. at different temperatures), the oil you put in your engine is made from about 80% base oil and 20% additives.
In the case of so-called "dino oils" the base stock is refined from crude oil extracted from the ground. In the case of synthetic oils, the base stock is chemically synthesized. Base stocks typically have molecular weights ranging from 300-600, whereas additives such as viscosity modifiers are very large polymers with molecular weights of 10,000 or more.
Why is it important?
Well, the obvious answer can be gleaned from looking at the above list of jobs the oil has. We wouldn't want to be lacking any of these in our engine.
In addition, and particularly important in high performance applications, using the proper oil with the correct physical and chemical properties for the application ( matched to the engine and operating conditions) minimizes friction and thus increases available power output and minimizes engine wear.
The specific physical and chemical properties come from the "formulation" of the oil - that is, from the specific combination of base stocks and additives.
Base Stocks
A variety of different processes are used in the manufacture of base stocks for engine oils. They include:
- Distillation
- Solvent refining
- Hydrogen processing
- Oligomerization
- Esterification
- Recycling & Re-refining
- Synthesizing
We're not going to go into these methods and talk about hydrocracking, converting aromatics to napthenes, isomerization, and the rest of the organic chemistry involved. While it may be interesting to some - it doesn't really mean much to we users because you can't usually buy an oil based on the method by which the base stock was refined.
Additive Packages
It is far more important for us to understand a little about additives in the oil - why they are required and what they do, because it is possible to do your research and buy certain oils based on their additive packages. This is true to a point, however people often way over-state the importance of a single additive, without realizing that in the complex polymeric machine that is modern oil - it is the combination, the whole package, that is important (and not just "buy brand X because it has a lot of additive Y in it").
A lubricant additive is a material designed to enhance the performance
properties of the base stock or to impart to the base stock properties that do not naturally exist within the base
stock. These additive agents are used at concentration levels ranging from several parts per million to 10 percent by volume or more.
Generally, additives are materials that have been chemically synthesized to provide
the desired performance features but some are naturally occurring materials that have undergone only minor
modifications.
Additives can carry out their task of enhancing or imparting new properties to an oil in one of three ways:
- Protection of engine surfaces. Engine protectors
include seal swell agents, antiwear agents, antirust agents, corrosion inhibitors, detergents, dispersants, and
friction modifiers.
- Modification of oil properties. Oil modifiers include pour point depressants, antifoam agents, and viscosity index (VI)
improvers.
- Protection of base stocks. Base stock protectors include antioxidants and metal deactivators.
Some additives possess
multifunctional properties.
It is an important consideration for oil manufacturers to use additives that are compatible with each other as well as with the base stock. From the consumer's perspective, it is equally important to use engine lubrication products that are compatible. The best way to achieve this is to:
- Not mix different types, brands or grades (viscosities) of oil in the engine.
- Not use any aftermarket additives in the oil - the manufacturer has already spent considerable effort engineering the optimum additive package for the oil and if you have chosen the right oil for the application, no extra additives are necessary.
Definitions
CLOUD POINT
The cloud point of a moisture-free oil is defined as the temperature at which
a cloud or haze appears in the lower portion of the test oil when tested (i.e., cooled) by ASTM D 2500. The
haze indicates the presence of some insoluble fractions, such as wax, at the temperature noted. In most
applications, this haze will have little practical significance.
POUR POINT
The pour point of an oil is defined as the lowest temperature at which the oil can be poured when tested by
ASTM D 97. The pour point can be directly related to whether or not the oil can be poured from a container
at low temperatures. Although pour point is a simple measure of wax crystal structure and low temperature
viscosity, more precise and correlatable viscometric methods, such as ASTM D 3829 and ASTM D 4684,
have been developed which better predict the ability of an oil to flow to the oil pump and throughout the
system at low temperature.
Viscosity
The most critical of an oil's properties is its viscosity, and how that viscosity varies with temperature, shear rate and pressure. The variation of lubricant viscosity with temperature, shear rate, and pressure is crucial to how the lubricant performs in an engine.
These properties are important in controlling oil film thickness and friction in the bearings, the piston assembly, and the valvetrain.
(Shear rate is the stress or force put on the oil molecules by sliding metal surfaces that tends to shear or break-up the molecular chains.)
The goal is to maintain correct/optimum oil film thickness (to separate the moving parts in the engine) while minimizing engine friction and therefore power losses. The oil must be able to do this under widely varying conditions of temperature and rpm/load, while remaining pumpable at low temperatures when cranking/starting the engine. No small task!
While the physical and chemical properties of an oil at operating temperatures (such as viscosity) are not related to oil
performance in a simple way, these individual properties are meaningful and are related to the oil's ability to
fulfill its function as a lubricant.
Viscosity is defined as the internal resistance to flow of any fluid. It is commonly thought of as how "thick" or "thin" the fluid is, or how "pourable" it is. For example, at room temperature, molasses has a very high viscosity, while water has a fairly low viscosity.
Viscosity is expressed as:
Dynamic Viscosity = (
Force / Sheared Area) / (Velocity / Film Thickness) = Sheer Stress / Shear Rate
The unit of measure for dynamic viscosity is the millipascal second (mPa·s), although the centipoise (cP) is
also commonly used. One mPa·s equals 1 cP. Oils that exhibit a constant viscosity at all shear rates in this
equation are known as “Newtonian” oils. Most single grade oils without additives are in
this category at temperatures above their cloud point. We don't generally use oils such as these in engines because, having a constant viscosity, they are either too viscous (thick) to be pumped when cold or too "thin" to provide adequate oil film when heated to operating temperature.
Oils that exhibit a viscosity which varies with changing shear rates in this equation are known as “non-
Newtonian” oils. Multi viscosity graded oils formulated with polymeric additives are generally in this category.
Almost any oil you put in your engine is going to be a non-Newtonian oil.
Another way you may see viscosity expressed is by Kinematic Viscosity. Kinematic Viscosity is determined by measurement using a method where the result is directly affected by the density of the liquid at the temperature the measurement was made. This method is easier than determining all the variables needed for the equation to calculate dynamic viscosity (above), but has the disadvantage that results are less directly comparable because of the effect of density. As such, strictly speaking it is best to either measure dynamic viscosity or to measure kinematic viscosity and density of the liquid at the temperature of
interest and convert kinematic viscosity to dynamic viscosity using the equation:
Kinematic Viscosity= Dynamic Viscosity / Density of Liquid
The unit of measurement for kinematic viscosity is the millimetre squared per second (mm^2/s), although the centistroke (cSt) is
also commonly used. One mm^2/s equals 1 cSt.
What does all this mean? It means that the viscosity of an engine oil changes with both temperature and with the load we place on it in the engine (pressure and shear rate). Since temperature, pressure and and shear rate can all vary widely in the engine (for example engine oil may be at 30°F when the engine is cold and first started, but rise to 300°F under hard use), we need a way to both identify and control (as much as possible) the viscosity of the oil under different conditions, because the viscosity plays such a huge role in achieving our goal, which we said was to maintain correct/optimum oil film thickness (to separate the key moving parts in the engine) while minimizing engine friction and therefore power losses. For an engine oil, the viscosity must be sufficiently
high so that the phenomenon of hydrodynamic lubrication can occur. This is where the flow of the oil forces the
bearing surface to ride on a thin film of oil and, thus, protect the lubricated surface from wear. Neither identifying the viscosity of an oil under different conditions nor controlling it is a simple task.
Before we move on, let's have a look at a simplified chart of some typical engine oil viscosities in cSt to give us some perspective:
SAE Motor Oil Grade |
Kinematic viscosity at low-shear-rate, in cSt at 100°C (212°F) |
30 |
10.3 |
0W20 |
8.3 |
5W30 |
9.9 |
10W30 |
10.8 |
15W40 |
14.9 |
20W50 |
17.8 |
Flow & Oil Pressure
What does all this complicated talk of viscosity translate to in the real world? The viscosity of the oil directly affects its pressure and flow as it is pumped around the engine to do its many jobs. Time to dispel a huge misnomer about engine oil and lubrication. Many folks mistakenly believe that oil pressure is the be-all end-all of engine lubrication - and understandably - as we have an oil pressure gauge after all. Unfortunately, this is not quite true. It is in fact the flow of oil that lubricates, cools, and cleans the engine. Adequate pressure to create this required flow is vital, to be sure. But do not be mislead into thinking that pressure is everything and the higher the oil pressure the better. In fact, pressure and flow are inversely proportional - as one goes up, the other must go down. So, for example, if you were to use a very thick (viscous) oil in your engine - pressure would go way up, but flow would go way down (it is, in fact, the resistance to the flow of the thick, viscous oil that causes the pressure to go up). The reason we have an oil pressure gauge is that it is much easier to measure oil pressure than oil flow.
So - adequate pressure is critical - but too much can be as bad as too little if it's an indication that the oil is too thick to flow adequately for lubrication and cooling. Imagine you used 90W/140 gear oil in your engine - a very thick, viscous oil. You'd have tremendous oil pressure - for a short while, anyway! But the oil would not flow enough to cool and lubricate and the engine would soon be destroyed. We'll return to this topic a little later when we discuss selecting and engine oil, but for now, keep in mind that oil pressure is vital, but not at the expense of flow - and that too much pressure is often a result of too little flow - more is not always better when it comes to oil pressure!
The golden rule for almost any engine is 10 psi oil pressure per 1000 RPM.
Viscosity Variation
Viscosity decreases rapidly with increasing temperature. For most oils, the
relationship between viscosity and temperature can be approximated by the empirical relationship
in the following equation:
loglog(kinematic viscosity+ 0.7 ) =A+B (log absolute temperature)
where:
A and B are constants, specific for each oil.
This relationship, which is an approximation of the MacCoull, Walther, Wright equation, forms the basis for
special viscosity temperature charts published in ASTM D 341. These charts permit the plotting of viscosity-temperature
data as straight lines over the temperature range in which the oils are homogeneous liquids.
The slope of these lines is a measure of the change in viscosity with temperature. It is dependent on the
chemical composition of the oil and is described by an empirical relationship called the Viscosity Index or VI. The higher the VI, the
smaller the change in viscosity with temperature (slope). ASTM D 2270 is used to determine VI values.
ASTM DS 39b is based on ASTM D 2270 and allows for more convenient determination of VI.
The Viscosity Index is calculated as such:
VI = 100+(10^(LN((EXP(1.0727+0.6175*LN(cSt@100)+0.9744*(LN(cSt@100)^2)+
(-0.3764)*(LN(cSt@100)^3)+0.04824*(LN(cSt@100)^4)))/cSt@40)/LN(cSt@100))-1)/0.00715
I warned you it was a complicated topic - didn't I?!
Let's try and simplify things a bit.
For engine oils, a relatively small change in viscosity with temperature (high VI) is desirable to provide a
wider range of operating temperatures over which a given oil will provide satisfactory lubrication. At low
temperatures, a relatively low viscosity oil is desirable to permit adequate cranking speed during starting,
and then adequate flow to the oil pump and the entire engine oiling system after starting.
At high temperatures in a running engine, the oil viscosity must be high enough to maintain adequate film
thickness between rotating or rubbing parts to minimize wear. Using a higher viscosity oil generally reduces
oil consumption (past piston rings and valve guides) and blowby, but increases friction associated with oil
film shearing in the piston/piston ring cylinder wall interface and in the bearings.
To extend the upper temperature limit at which an oil will still provide
satisfactory lubrication, polymeric additives, called Viscosity Index (VI) improvers, are widely used. Engine
oils properly formulated with VI improvers generally contain lower viscosity base stocks which provide better
low temperature cranking/starting and pumpability properties. As the oil temperature increases, the viscosity
of the oil containing a VI improver decreases more slowly than the same oil without a VI improper, thus
increasing the VI. The result is an oil that can give good starting/pumping response and is also effective in
providing a more viscous oil film at operating temperatures than could be obtained with a single grade oil
providing equivalent startability at low temperatures.
Oils containing a polymeric VI improver exhibit a decrease in viscosity as the shear rate or stress is
increased. Because the viscosity of such oils depends on shear stress, they are called “non-Newtonian oils.”
Such change generally lasts only as long as the oil is operated under such high shear stress. When the
shear stress is relieved, the oil reverts to its previous viscosity. This reversible decrease in viscosity due to
shear is called “temporary shear (or viscosity) loss.” When certain critically high shear stresses are imposed
on a VI improver in oil solution, the viscosity contribution of the VI improper to both low and high shear rate
viscosity can be permanently reduced. This nonreversible reduction in viscosity is called “permanent shear
(or viscosity) loss.” The magnitude of these temporary and permanent losses is dependent on the type and
molecular weight of the VI improver used, as well as the actual service conditions. In other words - VI Improvers can wear out.
Note that, in addition to VI Improvers, other chemical
additives, fuel dilution, contaminants from within and outside of the engine, wax in the oil, oil oxidation,
volatilization, and many other materials found in or added to the oil affect the viscosity. This is, of course, one of the reasons why even the best oils need to be changed regularly.
The use of VI Improvers is what gives us the "multi-grade" oils we are so used to today. Sadly, no oil is labelled with its VI, and the SAE labelling system in use today is actually a fairly broad system of classification which, if not well understood, can cause a lot of confusion and misunderstanding.
Let's have a look at it now.
SAE Standard Oil Labelling System.
So what does the labelling on the can, like "5W30", really mean and what does it tell us?
First and foremost, we must understand that the labelling on an oil can IS NOT telling us the viscosity of the oil - it is telling us the "Grade" of the oil according to the methods published by the SAE. That is, the SAE grade is a classification or grouping of oils based on viscosity, but not a measurement or statement of the oil's actual viscosity. In other words - the actual viscosity of oils of the same grade can vary - and sometimes by quite a bit.
Let's take a step back first, and look at things from the engine designer's perspective.
OK, if we refer back to our first chart of typical viscosities, we see that a straight 30 weight oil has a viscosity of about 10 cSt at 100°C. Simple then - the designer builds the engine and simply tells the user to use 30 weight oil. Done. What's all the fuss?
Not so fast grasshopper - remember all the things we just discussed that affect viscosity - chiefly temperature?
Our simple plan of "just use 30" would work fine if the engine and oil were always at a constant 100°C, and if it operated such that there were no changes in shear rate and pressure, nor any contaminants ever introduced. Alas, we know this is not the case. In the real world, we must contend with all these things, the biggest being temperature.
As we discussed, the viscosity of oil varies with its temperature. A straight "30”weight" oil may have the desired viscosity at 100°C (212°F), but look how it varies with temperature:
Temp ( °F ) |
Actual viscosity (cSt) |
Conditions |
Notes |
302°F |
3 |
Typical oil temp under racing conditions. |
Too thin to provide adequate hydrodynamic lubrication (metal-metal separation) |
212°F |
10 |
Typical oil temp under "Normal" operating conditions. |
OK |
104°F |
100 |
Typical oil temp at start-up, hot summer day. |
Too thick to pump and flow. |
32°F |
250+ |
Typical oil temp at start-up, winter day. |
Far too thick - will not pump or flow at all, engine will not crank or start. |
Clearly, as the temperature varies from the designed operating temperature the oil become unsuitable. As the engine heats up under hard use, the oil thins unacceptably and will not lubricate sufficiently. As the engine cools down to ambient temperature, the oil thickens unacceptably and will neither lubricate sufficiently, nor pump and flow sufficiently. Straight 30 weight oil has a very low Viscosity Index (VI).
The Solution?
While not perfect, the solution to this dilemma lies in the use of so-called "multi-grade" oils that do not exhibit the same change in viscosity with temperature as the good old straight 30 weight oil does. This is achieved either by adding VI improvers to mineral-base oils, or by the actual molecular synthesis of synthetic-base oils. The idea being to create an oil that neither thickens too much when cooled, nor thins too much when heated - but rather remains as close as possible to the ideal viscosity throughout the range of temperatures in which it is to be used.
DO NOT confuse multi-grade with multi-viscosity. Remember, the goal is to produce an oil that has a near-constant viscosity regardless of temperature (as much as is possible). Oils are assigned grades based on a number of criteria. In order to understand multi-grade oils, we must first understand single-grade oil classifications.
Single Grade Oils
There are two different groups or series of grades used by the SAE to classify engine oils. The difference between the series is the type of testing done on the oil.
The low-temperature-use or "W" grade oils:
In one series, the grade an oil is assigned is based on maximum low-temperature cranking viscosity, maximum low-temperature pumping viscosity, and a minimum kinematic viscosity at
100 °C. Oils in this series are identified by a number (often referred to as the "weight" of the oil") followed by the letter "W" (e.g. "10W"). Many folks believe the W stands for "winter use", presumably because these oils go though low-temperature testing. In fact, the W doesn't stand for "winter", but is just the method chosen to identify the series of oil grades that include low-temperature (below-freezing) testing - so it's easy to see why the connection was made between W and "winter".
SAE
Viscosity
Grade |
Low-Temperature Cranking
Viscosity (mPa·s)
Max |
Low-Temperature Pumping
Viscosity (mPa·s)
Max
(with no Yield Stress) |
Low-Shear-Rate
Kinematic
Viscosity (mm2/s)
at 100 °C
Min |
0W |
6200 at –35°C |
60 000 at –40°C |
3.8 |
5W |
6600 at –30°C |
60 000 at –35°C |
3.8 |
10W |
7000 at –25°C |
60 000 at –30°C |
4.1 |
15W |
7000 at –20°C |
60 000 at –25°C |
5.6 |
20W |
9500 at –15°C |
60 000 at –20°C |
5.6 |
25W |
13 000 at –10°C |
60 000 at –15°C |
9.3 |
Note that the figures in the table are LIMITS, either minimum or maximum, as indicated, that an oil must meet in order to be classified into that grade. They are not actual SPECIFIC guaranteed specifications. This is one reason why the performance of identicaly classified (and therefore labelled) oils can vary.
Column 1, Low-Temperature Cranking
Viscosity, correlates with the ability of an engine to start at low temperatures. Thus, for example, if you plan to start your engine when temperatures are colder than –25°C, you would need to choose a 5W or 0W grade oil.
Column 2, Low-Temperature Pumping
Viscosity, is a measure of an oil's ability to flow to the engine oil pump and provide adequate
oil flow during the initial stages of operation.
Don't ask me why the temperatures don't correlate between columns 1 and 2 - I don't know and it doesn't make sense to me why not - take for example the criteria for a 10W grade oil - it's hardly likely that you'd want to start an engine at only –25°C but run it at –30°C. (This is actually a sneak-peek at one of the conclusion I shall eventually draw - that the SAE labelling on the can doesn't really tell the average user a whole heck of a lot!)
Column 3, Low-Shear-Rate
Kinematic
Viscosity, has been shown to be related to certain forms of oil consumption (e.g. if kinematic viscosity is too low, the engine may suffer excessive oil consumption past the rings) and
have been traditionally used as a guide in selecting oil viscosity for use under normal engine operating
temperatures.
Obviously, since the criteria in the three columns are maximums and minimums, it is possible for an oil's actual test results to satisfy the requirements of more than one W grade. In labelling a W grade, only the lowest W grade satisfied may be referred to on the label. Thus, an oil meeting the
requirements for SAE grades 10W, 15W, 20W, and 25W, must be referred to as an SAE 10W
grade oil.
An oil must meet the pumping requirements of the lowest W grade satisfied by the cranking viscosity. If
the W grade defined by the pumping viscosity is higher than the lowest grade satisfied by the cranking
viscosity, the oil does not meet the requirements of SAE J300 and is, therefore, inappropriate for use.
If the kinematic viscosity at 100°C of an oil does not meet the requirements of the lowest W grade satisfied by the
cranking viscosity, then the oil does not meet the requirements of SAE J300 and is, therefore,
inappropriate for use.
The high-temperature-use grade oils:
In the second series, the grades are defined by a number only. In order to be assigned one of these grades, an oil must meet certain different criteria. Oils are assigned one of these grades based on their meeting a set of minimum and maximum kinematic
viscosities at 100 °C, and a minimum high-shear-rate viscosity at 150 °C.
SAE
Viscosity
Grade |
Low-Shear-Rate
Kinematic
Viscosity (mm2/s)
at 100 °C
Min |
Low-Shear-Rate
Kinematic
Viscosity (mm2/s)
at 100 °C
Max |
High-Shear-Rate
Viscosity (mPa·s)
at 150 °C
Min |
20 |
5.6 |
<9.3 |
2.6 |
30 |
9.3 |
<12.5 |
2.9 |
40 |
12.5 |
<16.3 |
3.7 |
50 |
16.3 |
<21.9 |
3.7 |
60 |
21.9 |
<26.1 |
3.7 |
Columns 1 & 2, Low-Shear-Rate
Kinematic
Viscosity, as for the W grade oils, has been shown to be related to certain forms of oil consumption and
have been traditionally used as a guide in selecting oil viscosity for use under normal engine operating
temperatures.
Column 3, High-Shear-Rate
Viscosity measured at 150 °C, is widely accepted as a
rheological parameter which is relevant to high-temperature engine performance. In particular, it is
generally believed to be indicative of the effective oil viscosity in high-shear components of an internal
combustion engine (for example, within the journal bearings and between the rings and cylinder walls)
under severe operating conditions. While the specific temperature and shear rate conditions experienced
by an oil in a particular application depend on mechanical design and operating parameters, the
measurement conditions specified are representative of a wide range of engine operating
conditions.To insure that polymer-containing oils do not create a situation in which the viscosity of the oil decreases
to less than a specified limit, minimum values of high-shear-rate viscosity are assigned to each of the
non-W viscosity grades as per column 3.
Multi-Grade Oils
So, let's return to our theoretical engine that we have designed to run at a water and oil temperature of approximately 100°C (212°F) and that requires an oil that has a kinematic viscosity of 10 cSt at this temperature.
Let's assume further that we need to be able to start and run this engine at temperatures down to -30°C.
Examining the SAE grade tables shows us that no single-grade SAE oil, whether W or non-W, will satisfy our requirements - the same conclusion we reached when we looked at the properties of straight 30 grade oil.
Enter the multi-grade oil.
In order to be classified as a multi-grade oil, and be assigned both a low and high-temperature SAE grade, an oil must meet the low-temperature cranking and pumping viscosity requirements of one of the W grades and all of the requirements of one of the non-W grades.
Depending on which combination of grades it meets, and subject to the requirement that only the lowest W grade satisfied may be referred to on the label, an oil may be assigned a multi-grade designation, such as 10W-30. In this case, the the W grade must precede the non-W grade, and the two grades must be separated by a hyphen.
If this is the case, it means we have an oil that satisfies both low-temperature performance requirements as well as high-temperature, high-shear requirements.
Many folks will refer to a multi-grade oil such as 10W-30 as an oil that "acts like a 10-weight oil when cold, and then acts like a 30-weight oil when hot". By looking at the tables above, we can see that this is not strictly accurate. All it means is that any oil so labelled meets the requirements of both a 10W oil and a 30 oil. The precise change of that oil's viscosity with temperature can only be achieved by plotting the Viscosity Index - something few if any users are ever going to do. That said, it's not entirely inaccurate to think of a multi-grade oil in this manner.
What we can say for certain is that a multi-grade oil will do a much better job of being thin enough at low or cold start-up temperatures to crank, pump, and flow well, while remaining thick enough at high temperatures to lubricate properly and provide the necessary hydrodynamic separation of metal parts.
By combining the requirements of the W and non-W grades into a single table it is easy to compile a table of requirements for any multi-grade oil. Here are some of the more popular multi-grades available on the market:
SAE
Viscosity
Grade |
Low-Temperature Cranking
Viscosity (mPa·s)
Max |
Low-Temperature Pumping
Viscosity (mPa·s)
Max
(with no Yield Stress) |
Low-Shear-Rate
Kinematic
Viscosity (mm2/s)
at 100 °C
Min |
Low-Shear-Rate
Kinematic
Viscosity (mm2/s)
at 100 °C
Max |
High-Shear-Rate
Viscosity (mPa·s)
at 150 °C
Min |
0W-20 |
6200 at –35°C |
60 000 at –40°C |
5.6 |
<9.3 |
2.6 |
0W-30 |
6200 at –35°C |
60 000 at –40°C |
9.3 |
<12.5 |
2.9 |
0W-40 |
6200 at –35°C |
60 000 at –40°C |
12.5 |
<16.3 |
2.9 |
|
|
|
|
|
|
5W-20 |
6600 at –30°C |
60 000 at –35°C |
5.6 |
<9.3 |
2.6 |
5W-30 |
6600 at –30°C |
60 000 at –35°C |
9.3 |
<12.5 |
2.9 |
5W-40 |
6600 at –30°C |
60 000 at –35°C |
12.5 |
<16.3 |
2.9 |
5W-50 |
6600 at –30°C |
60 000 at –35°C |
16.3 |
<21.9 |
3.7 |
|
|
|
|
|
|
10W-30 |
7000 at –25°C |
60 000 at –30°C |
9.3 |
<12.5 |
2.9 |
10W-40 |
7000 at –25°C |
60 000 at –30°C |
12.5 |
<16.3 |
2.9 |
|
|
|
|
|
|
15W-40 |
7000 at –20°C |
60 000 at –25°C |
12.5 |
<16.3 |
3.7 |
15W-50 |
7000 at –20°C |
60 000 at –25°C |
16.3 |
<21.9 |
3.7 |
|
|
|
|
|
|
20W-40 |
9500 at –15°C |
60 000 at –20°C |
12.5 |
<16.3 |
3.7 |
20W-50 |
9500 at –15°C |
60 000 at –20°C |
16.3 |
<21.9 |
3.7 |
Note: A special situation exists regarding the SAE 40 grade. Historically,
SAE 0W-40, 5W-40, and 10W-40 oils have been used primarily in light-duty engines. These multigrade
SAE 40 oils must meet a minimum high-temperature, high-shear-rate viscosity limit of 2.9 mPa·s.
In contrast, SAE 15W-40, 20W-40, 25W-40, and 40 oils have typically been used in heavy-duty engines.
The manufacturers of such engines have required high-shear-rate viscosity limits consistent with good
engine durability in high-load, severe service applications. Thus, SAE 15W-40, 20W-40, 25W-40, and
single-grade 40 oils must meet a minimum high-temperature, high-shear-rate viscosity limit of 3.7 mPa·s.
Of course, you cannot, and should not, use this table as the only method to selecting a grade of engine oil for your application. There is a great deal that isn't shown in the table - all the variables we've already discussed. All it shows is the criteria that must be met for an oil to be assigned an SAE grade. For example, if you look at the table, you could conclude that the only difference between a 5W-30 and a 10W-30 is that the 5W-30 has better cold start capabilities. While this is certainly true, it isn't the whole story. Consider that for both, the Kinematic Viscosity requirements at 100°C (normal operating temperature) are a range - from 9.3 - 12.5 cSt. It may be reasonable to assume that the 5W-30 may have a lower actual viscosity at this temperature, and probably at all temperatures below this.
When we recall that manufacturer's generally recommend oils based on the required viscosity at operating temperature, and we recall that all oils will be thicker than this at start-up (even if ambient temperatures are well above freezing) we may decide that 5W-30 is a better choice than 10W-30, even if we have no intention of operating at below-freezing temperatures, as it's viscosity will be less than the 10W-30 and therefore closer to the optimum viscosity, even as the engine is warming up to normal temp.
Mineral vs Synthetic
The method by which mineral and synthetic oils achieve multi-grade capability is different. Remember, multi-grade doesn't mean multi-viscosity - quite the opposite, it means the greatest possible ability to maintain a constant viscosity with changing temperature.
Mineral oils achieve this performance by adding VI Improvers that prevent the oil from thinning too much as temperatures increase. For example, a 10W-30 multi-grade mineral-based oil is made from a 10 weight oil and has VI improvers added to prevent it from thinning as much as a straight 10-weight oil when the engine reaches operating temp so that it has the properties of a 30 weight oil when hot.
In contrast, a 10W-30 multi-grade synthetic oil is based on a 30 weight oil. Because of this, no VI improver is needed as the oil will have the correct desired viscosity in an engine at normal operating temperature of 100°C (212°F). The difference is, because of the way a synthetic oil is manufactured using custom molecular structures, a synthetic oil can be produced that meets the requirements of a 10 weight oil when cold while also meeting the requirements of a 30 weight oil when hot. And since they are able to achieve this without VI improver additives, there are no additives to wear out as the oil is used. This is one of the advantages of a synthetic oil. By design they are less thick at start-up temperatures than mineral-based oils - even between oils with the same SAE grade.
This is one of the limitations of the SAE grading system - we can't tell from it that identically labelled oils can have dramatically different properties, including viscosity. However, much research, testing, and comparisons have been done and we know conclusively that a 10W-30 synthetic oil will have lower actual viscosity and better pourability, pumpability, and lubricating ability at cold temperatures than an identically graded 10W-30 mineral-based oil - even though they both qualify for the same SAE grading and labelling.
Without getting into any more complicated chemistry, other properties of synthetic oil make it a superior choice for engine lubrication, resulting in less wear, less friction, cooler running, and better power and economy. It is the all-round better choice except when breaking in a newly built or re-built engine where its superior lubricating properties can actually interfere with proper bedding in of the piston rings and cylinder.
But the main advantage that the synthetic has over the mineral based oil is the ability to lubricate at startup. Synthetics have lower viscosities and much better fluidity as the temperature drops (regrdless of their labelled grade). If temperatures get cold enough, mineral based oils eventually become unpourable and unpumpable - whereas synthetics can be used at much lower temperatures - this is why there are no 0W-xx graded mineral-based oils.
Engine vs Gear Oils
Before we leave the topic of oil labelling and SAE grades, let's just make one other point. Not all oils use the same grade structure. For example, gear oils use a different scale altogether from engine oils, and so the numbers are not directly comparable, even though they look very similar. For example, a 75W-90 gear oil has about the same viscosity as a 10W-40 or 10W-50 engine oil! The following table offers a rough comparison of different oil grades.
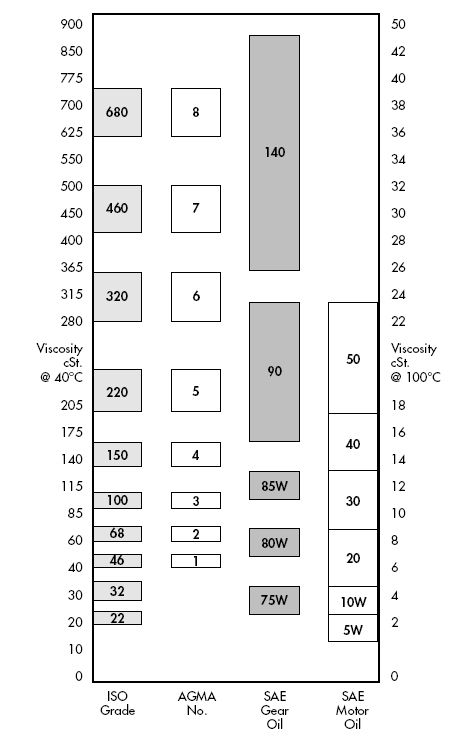
So - at this point you are probably about as sick of reading about oil viscosity as I am of writing about it. And yet we haven't come to any iron-clad conclusions or solid rules on choosing engine oil viscosity. About the only thing that we know for sure is that the detailed science and chemistry involved is WAY complicated. This may lead to the one best conclusion we can draw being: "deviate from the manufacturer's or engine-builder's recommendations with caution." Hopefully, what we have accomplished though is to at least have increased our understanding of the subject so that we are better able to evaluate further research we do or advice we are offered. For example, if you read on some forum some piece of advice or opinion about what to use - now you can evaluate that from at least a semi-educated perspective. This might just be the most useful lesson to take away from all this.
Choosing an Oil
OK, so we have learned that selection of the optimum oil:
- Ensures adequate cold start cranking, pumpability, and flow.
- Minimizes engine friction (and thus maximizes available power).
- Protects against engine wear (providing the optimum oil film thickness for the engine design and service conditions).
- Provides adequate flow for cooling and lubrication while maintaining suitable oil pressure throughout the operating range (temperature, speed, load etc.) of the engine.
- Maintains the fine line between minimizing friction and maximizing lubrication, as the two are somewhat at odds, most notably in the bearings (main and con-rod), piston assembly, and valvetrain.
There are also other properties and qualities the optimum oil must have, with most of these related to the additive packages used in the oil's formulation. For example, oil must also have good anti-foaming performance (esp. in high rpm applications) because aerated oil can cause problems with hydraulic actuation (for example, in hydraulically activated valve lifters) - like air in the brakes. Also, aerated oils can cause cavitation damage in bearings.
However, the bottom line is, none of the the physical and
chemical tests described in any of the many ASTM, API, or SAE documents listed in the references section can be used effectively for accurately defining performance characteristics at operating loads and
temperatures. Only actual performance evaluations in special laboratory engines or in actual field tests will define
the capabilities of an engine oil.
What that means is that reading an oil's specification, examining the formulation, pouring over the list of additives, arguing over the brand or type - none of these things will actually determine how an oil will perform in any particular application. Only laboratory testing, dyno testing, and real-world testing can determine this
Although laboratory engine tests like those described in SAE J304 are necessary and valuable aids to engine oil development and
evaluation, they have limitations. In many instances, the final proof-of-performance is established by field tests
of the oil in actual vehicle service.
Although oil performance in the engine is related to
base stock and additive composition, it is often difficult to assign a specific aspect of such performance totally
to the use of a specific additive or base stock. Part of the reason is that some of the physical and chemical
properties of the oil overlap in their influences on engine performance and durability and it is presently difficult
to directly and unambiguously attribute such effects to either the chemical or the physical properties of the oil.
Progress in developing this level of understanding is being made. Some of these performance characteristics
of engine oils are discussed in SAE J183, SAE J300, SAE J1423, and SAE J2227. At the present time, oil
physical and chemical properties can be related to engine performance and durability only with the guidance of
engine manufacturers and with appropriate and jointly accepted engine and/or field tests successfully
completed on that oil.
What does this mean for us?
It means that the truly dedicated looking for every ounce of performance are in for a LOT of research, testing, and evaluation. A good place to start is by reading the papers listed in the reference section at the end of this article, contacting and working with oil manufacturers and engine builders, and developing and performing relevant performance tests to be followed by analysis of used oil samples. That's a lot of work. Most of us will never have the resources to do this.
So where does that leave the rest of us? Fortunately, not hung out to dry. The lubrication requirements for modern engines are extremely complex. Current engine oils
are the result of extensive research and development aimed at meeting these requirements. Lubricant and additive
manufacturers, engine manufacturer organizations, the
Society of Automotive Engineers, Society of Tribologists and Lubrication Engineers, American Society of
Mechanical Engineers, American Petroleum Institute, American Society for Testing and Materials, etc. are all full of very smart folks whose life's work it is to produce the best engine lubricants possible - and they do a damn fine job. Today's oils are FAR superior to those available even a decade or two ago.
One of the most common and familiar methods for engine manufacturer's to specify suitable oils for their engines is by use of the API Engine Oil Licensing and Certification System. This is a system of engine oil performance categories and classifications developed through
the efforts of the Alliance of Automobile Manufacturers (AAM), American Petroleum Institute (API), the
American Society for Testing and Materials (ASTM), the Engine Manufacturers Association (EMA),
International Lubricant Standardization and Approval committee (ILSAC) and SAE.
It provides a communications tool for both
engine manufacturers and oil marketers to describe a complex series of performance specifications
to the user through a simple designation of several letters or symbols. This simplifies the oil
marketer’s job of communicating the applications for which his oil is suitable by using the same
designations on his containers. More importantly, the consumer can easily link the engine
manufacturer’s recommendation with the information on the oil marketer’s label to assist him in
selecting the proper oil for his engine.
The system embodies several different types of classifications including the API “S” series
which describes engine oil standards primarily for gasoline engine service, the API “C” series which
defines standards for diesel engines, and the ILSAC GF Series, “Minimum Performance Standard for
Passenger Car Engine Oils.”
The latest "S" series specification is "SM". This can be found on the back of an oil bottle or can in the familiar circular symbol, like this ("SJ" specification shown):
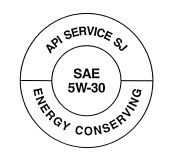
For those interested in further details of the API Engine Oil Licensing and Certification System, I recommend reading SAE J183 "Engine Oil Performance and Engine Service Classification
(Other than “Energy Conserving”)" and SAE 1423 "Classification of Energy Conserving Engine Oil for Passenger Cars,
Vans, Sport Utility Vehicles, and Light-Duty Trucks"
On the whole, engine oil performance improves with each successive specification in the series - for example, SH oils outperform SG oils. However, in some cases, engine oils with newer classifications may not always provide satisfactory performance where older
classifications are recommended. A recent example I have read about but don't have a lot of experience with is the recent reduction of ZDDP levels in the change from SL to SM oils. Basically, the most common additive package for motor oils designed to reduce wear (anti-wear) is a combination of zinc and phosphorous that is commonly called ZDDP. When ZDDP burns it produces ash that tends to be detrimental to catalytic converters and other emissions equipment, so levels of it have been reduced in SM oils, and replaced with other no-ash antioxidants. There's a whole lot of people out there pouring over spec sheets and how many parts per million of ZDDP there are in various oils - some claiming SM oils are the devil. As I said - I don't have a lot of personal experience with this topic - but a lot of what I have read doesn't seem to be backed up by a whole lot of solid science. Most of all, remember that:
Although oil performance in the engine is related to
base stock and additive composition, it is often difficult to assign a specific aspect of such performance totally
to the use of a specific additive or base stock. Part of the reason is that some of the physical and chemical
properties of the oil overlap in their influences on engine performance and durability and it is presently difficult
to directly and unambiguously attribute such effects to either the chemical or the physical properties of the oil.
At the present time, oil
physical and chemical properties can be related to engine performance and durability only with the guidance of
engine manufacturers and with appropriate and jointly accepted engine and/or field tests successfully
completed on that oil.
Rules of Thumb
After all this, I hope you weren't expecting me to be able to tell you exactly what the best oil to run in your engine is?
About the best I can do is offer you my opinions and thoughts on the matter in the form of some semi-educated rules-of-thumb.
- Choose a high-quality, name brand, synthetic oil meeting the latest API "S" series / JASO MA performance specification.
- Follow the manufacturer's or engine builder's recommendation for viscosity unless you really know what you're doing.
- Failing that - full synthetic 5W30 will do a good job in most engines.
- Most of us, even weekend racers, will not benefit from "racing oils" and in fact they can do harm if you're not doing full race tear-down maintenance, since many lack additives such as cleaners and dispersants.
- Always take what you read on the internet with a huge grain of salt - including this article I guess! (ironic, eh?). When researching, beware of the tenancy for people to justify their own decisions rather than share honest experience (including mistakes).
- Be aware of marketing influences - Ferrari may recommend nothing but Shell oils now - same for GM and Mobil - but those are big dollar marketing deals - it doesn't mean no other oil is as good. However, it does mean that you can probably trust that oil though - since no marketing deal is going to be good enough to get Ferrari to recommend a sub-standard oil that would cause damage in their engines.
- Change your oil frequently.
- Cool it. One of the best things you can do is add a quality oil cooler in high load, high rpm applications.
- Filter it well - use a quality aftermarket full-flow canister style filter.
- Don't expect too much of your oil - it already has a lot to do, under brutal conditions - keep it cool, clean, and filtered to give it a chance to do it's job. Do not expect a switch to a different oil to make up for worn rings or valves or leaky seals or gaskets - fix the problem then use the proper oil!
- Keep the entire oiling system well maintained - even the best oil in the world won't work well if you're upside down with a clogged filter, worn oil pump, leaky plumbing, and no accumulator.
- Err on the side of lighter oils. There has been a steady trend over the last couple of decades towards lighter and lighter oils, despite engine performance, heat, rpm, and power steadily increasing. Thirty or forty years ago, 300hp 4 and 6 cylinder engines were unheard of, as were 0W-xx oils. Today, however, it's is not uncommon for a small 4 cylinder engine to produce a couple of hundred horsepower, rev to 7 or 8 grand, possibly be turbo- or super-charged and be designed to use a 5W-20 oil. I see a clear trend where engines performance and demands are rising steadily, while at the same time recommended oil viscosities are falling.
- Closely related to the above point, treat with caution the advice of some of the old-timers. As experienced as they are - they cannot help but be influenced heavily by their early experience and teachings - and for many it can be quite a challenge to cast aside much of what they knew to be true in the past in favour of newer, highly complicated technologies such as those involved in the modern tribology and rheology of light viscosity synthetic oils. A guy who cut his teeth building old-school carbureted small block hot rod engines using 20W-50 mineral oils may have a hard time switching his thinking to all-aluminum fuel-injected V8's using 0W-30 synthetic oils.
- If you're truly dedicated to finding the optimum oil for you application, and have the time and money to do the research and testing, look into having oil analysis performed on your used oil. There are plenty of companies that offer a used oil analysis service for reasonable rates. Try a Google search for "used oil analysis" to get started. Regular and repeated teardown and inspection of the engine after controlled testing under consistently controlled conditions would also be required to produce the most thorough and reliable results. Oil performance could then be evaluated in terms of rust and
corrosion, sludge, varnish, piston ring zone deposits and condition, wear, bearing corrosion, fuel
efficiency, and peak power produced. Yea - that's a stupid amount of work. F1 teams, maybe NASCAR, they may do it - but probably few others.
|